USE OF COMMERCIAL AQUACULTURE FEED AND MICRODIETS INCORPORATING PROBIOTICS IN SHRIMP FARMING
White shrimp, is an economically significant species that dominates shrimp production and represents a rapidly growing industry. However, the culture faces challenges due to high mortality rates due to bacterial and viral diseases. Introducing probiotics into shrimp culture can enhance growth, survival rates, and immune responses against disease outbreaks caused by pathogenic bacteria or viruses. In recent decades, aquafeed has explored effective solutions, such as microdiets, co-feeding (combining commercial feed and probiotics), and various microdiets types, to address aquaculture disease problems. Combining microdiets containing essential nutrients with probiotics has proven to be safer than antibiotics, which can adversely affect animals and the environment.
INTRODUCTION
Crustaceans, particularly penaeid shrimp, play a vital role in the global fishery and aquaculture farming industry as significant economic resources. In 2018, the worldwide production of farmed shrimp increased by 3 to 5% from the previous year, reaching approximately 4 million tons (Manan et al. 2020). Pacific white shrimp (Penaeus vannamei) is widely distributed due to high consumer demand, abundant nutritional value, and high growth rate. With a high economic value, aquaculture provides a high-quality protein feed. However, fishmeal production resources have become inadequate for farmers due to El Niño, overfishing, and marine pollution (Chen et al. 2022). Other factors contributing to the challenges in sustaining shrimp production in Asia include disease outbreaks, poor management practices, and environmental degradation (Thakur & Lin 2003).
The increasing shrimp production relies on intensive aquaculture systems, which require substantial input, such as feed and shrimp stocks. High market demand, especially for P. vannamei, has led to serious problems, including parasites and bacterial infections (Jefri et al. 2020, Seonghun 2020). Shrimp species cultured in Asian countries belonging to the Penaeidae family include P. vannamei, P. monodon, P. japonicus, P. indicus, P. orientalis, and P. merguiensis (Shiau 1998, Samocha & Prangnell 2019).
P. vannamei has become a dominant species in global shrimp culture, thriving in extensive and bio-floc systems. In contrast, black tiger prawn (P. monodon) was the leading cultured species in Asia during the 1990s; however, its production declined due to viral diseases and economic impacts. The prominence of white shrimp has become more evident due to the availability of specific pathogen-free (SPF) broodstock (Samocha & Prangnell, 2019).
Aquaculture diseases present significant challenges to farmers raising and culturing aquatic species, including freshwater or marine fishes and invertebrates, and researchers studying these organisms through scientific methods. The aquaculture industry has grappled with bacterial, fungal, and viral issues traditionally managed using antibiotics and chemical disinfectants (Huang et al. 2016). Antibiotics and vaccines have limitations, such as promoting drug-resistant bacteria, causing residue in aquatic species and the environment, high costs, and restricted usage (Das et al. 2020).
High bacterial problems have led to substantial mortality and estimated economic losses of around US$ 0.49 billion from 2011 to 2014 due to acute hepatopancreatic necrosis disease (AHPND) (Kua et al. 2016). Recent research in Terengganu has identified cases of high mortality in P. vannamei linked to AHPND caused by Vibrio harveyi and V. parahaemo-lyticus (Amatul-Samahah et al. 2020a). Feed quality is crucial to the increasing demand for shrimp (Chen et al. 2021). However, intensive shrimp culture can result in environmental degradation and crop losses due to disease outbreaks, often attributed to high water exchange and effluent discharge (Xu et al. 2018).
Researchers have recently explored nutritional requirements in commercial shrimp feeds to meet growth performance, physiological development, maturation of internal organs, and resistance to pathogen infections. Feed formulation and quality can impact shrimp development and water quality (Xu et al. 2018). Overfeeding can lead to excess organic waste, resulting in imbalanced water parameters. Nutrient deficiency can also negatively affect shrimp growth, increasing competition, fin damage, and mortality (Hixson 2014).
Farmers have increasingly turned to alternative feed sources, such as probiotics, which are cost-effective and accessible. Probiotics, live microorganisms that provide host benefits when administered adequately, can help improve immune response, disease resistance, growth rate performance, and gut microbiota activities in shrimp. Microfeeds incorporated with probiotics have been researched, but studies on disease resistance are limited. This review explores the advantages of using feeds available for P. vannamei culture, specifically focusing on their role in promoting growth and enhancing disease resistance.
White shrimp (Penaeus vannamei)
White shrimp, P. vannamei (Boone, 1931), also known as Litopenaeus vannamei, is one of the main shellfish species reared worldwide besides black tiger prawn (P. monodon), which accounts for 52.9% of the world’s total aquaculture production of the crustacean species (FAO 2020, Seonghun 2020). P. vannamei is listed as the sixth most cultured species globally, with 5 billion tons produced in 2018. Based on statistical data from the Food and Agriculture Organization (FAO) retrieved from Tsai et al. (2019), the total shrimp production increased to 110.21 million metric tons in 2016 compared to the previous year (1105.46 million metric tons), with white shrimp being the most popular species. Shrimp breeding represents about 64% of total shrimp production, dominated by white shrimp (Camacho-Jiménez et al. 2020). In 2020, these species produced 5.4 million metric tons, primarily cultured shrimp species, and make up to 80% of the total aquaculture production of crustaceans (FAO 2022). This shrimp is cultured in intensive aquaculture systems in most Asian countries such as Malaysia, China, Thailand, and Taiwan (Manan et al. 2015) due to the high demand and shrimp marketing production, but easily susceptible to disease infections. Most of the common diseases that infect white shrimp comprise AHPND (Amatul-Samahah et al. 2020a), white spot syndrome virus (WSSV) (Bondad-Reantaso et al. 2005, Wang & Gu 2010), early mortality syndrome (EMS) (Manan et al. 2015), and vibriosis (Thompson et al. 2010, Nimrat et al. 2012). Thompson et al. (2010) and Cheng et al. (2021) stated that the immune system of shrimps is different from vertebrates because they rely on the innate immune system instead of a specific immune response due to the lack of lymphocytes, dendritic cells, macrophage, and molecular machinery, such as immunoglobulin.
Another one of the main penaeid shrimp species in aquaculture production is P. monodon, a black tiger prawn after white shrimp. This species is also constantly challenged by disease, especially Vibrio spp. (Temario et al. 2022). Since this shrimp is affected by the disease, the antibiotic treatment is no longer effective since it develop into disease resistance, and using probiotics is one alternative to avoid it. Besides using a commercial probiotic, the potential probionts isolated from the shrimp can also help inhibit against disease.
The main problem in shrimp aquaculture is the high mortality caused by bacterial infections, viral infections, poor water quality management, and inefficient culture systems. Many shrimp diseases have been studied by researchers worldwide. According to Thitamadee et al. (2016) and Lee et al. (2022) the list of diseases includes vibriosis, EMS, AHPND, infectious hypodermal and hematopoietic necrosis virus (IHHNV), black gill disease, hepatopancreatic haplosporidiosis (HPH), white spot disease (WSD), yellow head disease, white tail disease (WTD). This review covers certain dangerous diseases that have caused high mortality to the penaeid shrimps on the farm until now.
One of the primary causes of high mortalities of shrimps is AHPND, where the outbreak began in southern China in 2009 and then spread to Vietnam, Malaysia, Thailand, and Mexico from 2010 until 2013 (Thitamadee et al. 2016, Amatul-Samahah et al. 2020b, Schofield et al. 2021). In 2011, Malaysia experienced this outbreak that impacted the economy, causing a significant decrease in shrimp production to 67,000, 55,000, and 50,000 metric tons until 2013 (Amatul-Samahah et al. 2020b). The common penaeid shrimps susceptible to this disease include P. vannamei and P. monodon (Muthukrishnan et al. 2019). Some reports stated that the white shrimp, primarily infected by AHPND or vibriosis, suffered a significant loss with 100% mortality (Tran et al. 2013). This disease was initially described as EMS due to the new pattern and high mortality clusters during the early grow-out stages of P. vannamei and P. monodon (up to 100% within 30 days of post-stocking). A change was made when histological examination of the hepatopancreas of affected shrimp revealed massive sloughing of tubule epithelial cells and hemocyte aggregation with hepatopancreatic and bacterial septicemia by secondary bacterial infections (Thitamadee et al. 2016). Previous studies have shown that Vibrio species are involving other diseases such as red disease, luminous vibriosis, tail necrosis, loose shell syndrome, and white gut disease, which mainly infect other shellfish species, for example, lobster and crabs (Abdel-Latif et al. 2022). Tran et al. (2013) reported that isolates of V. parahaemolyticus were a causative agent of AHPND, which causes the sloughing of tubular epithelial cells. Furthermore, Lighner et al. (2012) also mentioned that this disease infects the hepatopancreas of shrimps. The physiological effects of the previously cited EMS appear to be limited, and the symptoms are as described by Zorriehzahra & Banaederakhshan (2015), whereby shrimps feeling lethargic, spiral swimming, anorexic (loss of appetite), hepatopancreatic discoloration (causes dark coloration of the shell) and mottling of the carapace.
Another pathogenic disease often occurring in penaeid shrimp culture is WSD, categorized as one of the most lethal diseases. This disease affects the industries in terms of overall production, emerging as an obstacle to sustainable shrimp production (Stentiford et al. 2012). WSD is highly contagious and can cause high mortality of up to 100% in sensitive hosts within 3 to 10 days post-infection and some 7 to 10 days (Peng et al. 2001, Dey et al. 2020, Govindaraju et al. 2020, Amar et al. 2021). This disease is caused by a pathogen known as WSSV. The pathogen is classified under the family Nimaviridae, genus Whispovirus, with a double-stranded DNA genome that varies in size from 292.96 to 305.1 kilobase pairs (kbp) (Sentiford et al. 2012, Tuyen et al. 2014, Amar et al. 2021). The manifested clinical symptoms include lethargy, loss of appetite, anorexia, white-reddish body coloration, and white spots or patches on the exoskeleton and carapace. However, in the case of acute infections, the signs were lethargy and a reduction in food consumption (Pradeep et al. 2012). Under some circumstances, white spots at the carapace are displayed long after the infection, reaching where the host (white shrimp) is on the verge of death (Dey et al. 2020). Other gross pathologies are discoloration of hepatopancreas, swellings on branchiostegites due to excessive fluid, low response to stimulus, and thinning and delayed hemolymph clotting (Hameed et al. 2003). This disease hampered the production and health of the penaeid shrimps. WSSV spreads from an infected host to other healthy shrimps through the water column into the gills and other body parts, feeding contaminated feeds and poor water quality. Stentiford et al. (2012) suggested that the transmission of WSSV is probably due to the importation of unscreened broodstocks. Peinao-Guevara & Lopez-Meyer (2006) reported that several rainy periods increased the prevalence of WSSV in white shrimp commercial ponds with changes in temperature and salinity. In Malaysia, WSD is more severe during wet than dry seasons (Oseko et al. 2006). Kakoolaki et al. (2011) suggested that changes in salinity increase the virulence of WSSV (from 30 to 50). Another mean is through horizontal transmission influenced by other biological factors, such as the virulence of WSSV strains, the transmission of the virus from one host to another, host density, and the age factor of the host causing different immune responses towards the virus, as well as predation and behavior of the shrimps (Venegas et al. 2000, Wu et al. 2001, Waikhom et al. 2006). Moreover, different host species may have a similar transmission rate (Dey et al. 2020). In contrast, other authors had different views on direct and indirect transmission rates in relative contribution (Tuyen et al. 2014).
Vibriosis is another major disease problem that occurs in shrimp culture worldwide. Vibriosis causes mass mortalities in shrimp culture during the adult or larvae stage, viral co-infection, and growth retardation. (Zubaidah & Yuhana 2015, Amatul-Samahah et al. 2020a). Martinez-Urtaza et al. (2016) describe this disease as epizootic, which happens temporarily and is widespread in the animal population. The main causative agents are V. harveyi and V. parahaemo-lyticus (Amatul-Samahah et al. 2020a). Vibrio species are ubiquitously found in marine aquaculture systems and environments and are the most prevalent bacteria that affect various marine fishes and shellfish (Ina-Salwany et al. 2019, Möller et al. 2020). Tepaamorndech et al. (2019) remarked that V. harveyi causes luminous vibriosis, white tail disease, and bright-red syndrome in shrimps. Some authors asserted that Vibrio are opportunistic pathogens affecting the host following fluctuations in water quality (temperature, salinity, and dissolved oxygen) (Aguilera-Rivera et al. 2019). The ability of Vibrio to cause diseases can be a complex process triggered by changes in physiological conditions, environmental stress, and strain pathogenicity (Anwar 2020).
Some clinical signs manifested in infected shrimps include pale or yellow coloration of the carapace, necrotic or pale hepatopancreas, melanization, lethargy, anorexia, and opaque abdominal muscles (Soto-Rodriguez et al. 2010, Haditomo et al. 2018). Another factor that could trigger a vibriosis outbreak is insufficient food, which causes stress in cultured species (Ina-Salwany et al. 2019). Some studies showed that V. harveyi is associated with other shrimp diseases with high virulence. Soto-Rodriguez et al. (2010) demonstrated that V. harveyi strain CAIM 1792 found in P. vannamei was the etiological agent of bright-red syndrome (BRS). Supporting this, Sun et al. (2008) revealed that the viable but non-culturable (VBNC) V. harveyi expresses its virulence characteristics when detected using a hemolysin gene. However, the bacteria temporarily loses its pathogenicity if there is no expression of the hemolysin gene, proving that V. harveyi can maintain its pathogenicity and virulence, which can be triggered under favorable environmental conditions. Muthukrishnan et al. (2019) documented that V. harveyi and V. parahaemolyticus caused AHPND in P. vannamei. Various diseases of penaeid shrimps, causative agents, and symptoms are compiled in Table 1.
The application of probiotics in shrimp culture for disease resistance
Probiotics are feed additives and effective supplements due to their effects to enhance the growth of the host, providing nutrients, improving immune responses, promoting gut maturation, improving metabolisms, improving water quality, boosting stress tolerance, and enhancing disease resistance (Farzanfar 2006, Selim & Reda 2015, Tachibana et al. 2020, Ringø et al. 2020, Monier et al. 2023). Probiotics are living microorganisms that confer beneficial health benefits when supplied adequately to the host. Various probiotics that enhance the immune system, growth performance, and survival of penaeid shrimps include Bacillus, Lactobacillus, Enterococcus, and Lactococcus (Kuebutornye et al. 2019).
Probiotics have been increasingly important in replacing antibiotics in shrimp culture to prevent adverse effects on environmental health (Babitha & Murugaian 2020). Many probiotic studies were undertaken on various aquaculture species, such as fish, shrimps, and mollusks, to understand their beneficial activities in the gut of the host, including their positive effects on growth performance and immune responses to diseases (Gu 2010, Wang & Gu 2010, Jefri et al. 2019, Mazón-Suástegui et al. 2020, Won et al. 2020).
The most commonly used probiotics in shrimp culture are lactic acid bacteria (LAB), Bacillus, Streptomyces, Vibrio, Pseudoaltermonas, Paeniba-cillus, and Pseudomonas (Li et al. 2007, Kewcharoen & Srisapoome 2019, Hazel 2020). Another probiotic that stimulates the immune response for the production of inhibitory substances against foreign antigens and is unaffected by antibiotics is yeast (Saccharomyces cerevisiae). Wahché et al. (2006) stated that yeast can colonize the gut and release digestive enzymes inside the host. Several research studies or experiments have been conducted on probiotics in shrimp culture to determine the gut content and disease resistance activities inside the host when being treated. One of the common probiotics widely used in aquaculture is Bacillus sp. (Zokaeifar et al. 2012). Bacillus subtilis is one of the most popular and widely used in the aquaculture industry (Jamali et al. 2015, Abdel-Tawwab et al. 2020). Lactobacillus sp. is also widely used in shrimp culture. Lactobacilli are gram-positive, rod-shaped, and non-spore-forming bacteria that modulate normal gut microbiota in animals (Naiel et al. 2021). A B. subtilis strain isolated from adult shrimp’s gastrointestinal tract (P. vannamei) promoted disease resistance against V. parahaemolyticus as in-vitro (Tank et al. 2018). Likewise, V. alginolyticus, Roseobacter gallaeciensis, and Pseudomonas aestumarina were also efficient probiotics to resist bacterial infections due to their antimicrobial activities against V. harveyi, V. vulnificus, and V. fluvialis (Ringø 2020), proving that by using a pathogenic bacterium is also one of the efficient ways against the most common bacterial disease infection in penaeid shrimp culture.
Moreover, some research used V. gazogenes mixed with chitin provided more health benefits to P. vannamei due to its effects on the Vibrio-like bacteria in the fore and hindgut, hepatosomatic index, and hemocyte numbers (Thompson et al. 2010). An investigation on the effects of ozone and probiotics (Bacillus S11) on the survival of P. monodon found that shrimp survival was significantly higher when challenged against V. harveyi (Meunpol et al. 2003). Psychrobacter sp. supplemented to the postlarvae of P. vannamei improved growth performance, total protein content, osmotic stress resistance, survival rate, gut activities, and disease resistance when challenged with Aeromonas sp. through immersion at 5×105 CFU mL−1, whereby the survival rate was 74.5% compared to untreated postlarvae at 47.1% (Franco et al. 2016).
Various reports have also proven the efficiency of probiotics in water quality management. Probiotics can improve water quality, inhibit pathogenic growth, and increase the growth of cultured organisms by decomposing organic materials in the water. Heterotrophic bacteria can remove nitrogenous compounds through heterotrophic denitrification by transforming total ammonia nitrogen (TAN) into beneficial microbial biomass (Abakari et al. 2021). Abdel-Tawwab et al. (2020) demonstrated that B. subtilis in fermented rice bran reduced the TAN and nitrite levels in P. vannamei culture. Furthermore, Wang et al. (2005) also revealed that commercial probiotics improved the water quality for the P. vannamei culture by significantly reducing the nitrogen and phosphorus concentrations in the pond.
Similarly, the application of either micro-encapsulated or freeze-dried probiotics (Bacillus sp.) through the enrichment of Artemia or water additive reduced ammonia, pH, and nitrite levels in the culture of penaeid shrimp (Nimrat et al. 2012). Nimrat et al. (2012) also proved that the application of probiotics via different modes significantly reduced the levels of ammonia, nitrite, and pH while increasing the ratio of Bacillus sp. in the culture water of P. vannamei postlarvae. The conversion of ammonia and nitrite into nitrate through nitrification reduces ammonia and nitrite levels. Since nitrification discharges hydrogen into the water body, it can decrease pH (Zhang et al. 2022). Therefore, probiotics can prevent fluctuations and improve water quality in shrimp culture, rendering better health and growth.
The beneficial effects of probiotics can be explained by their efficiency in reducing the cost of grow-out production and treating diseases compared to commercial feeds and antibiotics. However, there are many ways to administer probiotics in various forms to shrimp or fish, such as live food enrichment through the water body, micro-encapsulation, nano-encapsulation, freeze-dried probiotics, and incorporation into commercial feeds (Nimrat et al. 2011, Sumon et al. 2018). Table 2 compiles various probiotics, modes of administration, and their beneficial effects.
In a study, powder-formed probiotics were proven better than liquid-formed on shrimp cultures regarding water quality, growth performance, and the ratio of Vibrio sp. to total bacteria (Atmomarsono & Susianingsih et al. 2020). Another route of administration of probiotics to the guts of the host is through bio encapsulation with zooplankton, which increases zooplankton availability, growth, and reproductive capacities. Other methods include blending with food supplements or directly into the water column (Sumon et al. 2018). Babitha & Murugaian (2020) revealed that using a commercial feed incorporated with probiotics enhanced fish growth.
Commercial feed such as fish meal (FM) is essential in aquaculture because it provides sufficient nutrients to the culture species, aiding in growth development from larvae to adult, internal organ development, enhancing the immune system, avoiding nutrient deficiency in external and internal organs, conferring health benefits, and accounts for 25-35% in shrimp feed formula (Kuhn et al. 2016, Chen et al. 2021). FM is also considered an ideal protein source in aquatic animal feed agro-industry, and marine shrimp aquaculture agro-industry is among the top fish meal supply consumers (Chuchird et al. 2023). However, excessive feeds and deficiency of nutrients in some commercial diets cause an unbalanced nutrient cycle, poor water quality, and proliferation of unwanted microorganisms, which lead to the high susceptibility of cultured species to bacterial or virus diseases (Miller & Mitchell 2009). Ng et al. (2015) proved that an organic acid blend decreased the mortality of P. monodon up to 30% when challenged with V. harveyi.
The nutritional requirement of penaeid shrimps consists of protein (non-essential amino acids and essential amino acids), lipids, vitamins, minerals (zinc, calcium, iron, and manganese), carbohydrates, and energy (Kaushik & Seiliez 2010, Molina-Poveda 2016, Prangnell et al. 2022). Nutrient deficiencies negatively impact health, increasing disease susceptibility (Hixson 2014).
Protein is one of the most expensive nutrient components in the shrimp diet (Prangnell et al. 2022). Protein consumption is indispensable for shrimp because it continuously supplies all essential amino acids for body maintenance and growth (Kaushik & Seiliez 2010, Daniel 2018). Protein requirements in the diet should be considered and examined carefully due to specific requirements in different cultured species (Molina-Poveda 2016). Variations exist in the protein requirements of shrimps to achieve good health status. Protein is essential for shrimps (representing 65-85% of body weight) as their amino acid profile determines their quality (Molina-Poveda 2016). The amount of protein needed for shrimps must be at least what is required, as this will affect their growth performance (Prangnell et al. 2022). During the nursery phase, shrimps have a high requirement for protein to reach later stages (Correia et al. 2014, Samocha & Prangnell 2019). Therefore, knowing the optimal protein requirement for the host’s dietary energy supply is necessary.
Lipids serve as a source of energy and essential fatty acids (e.g. eicosahexaenoic and docosahexaenoic acids). Lipids also play an essential role as carriers of fat-soluble vitamins, such as vitamins A, D, E, and K, and precursors for hormones and metabolic regulators (Somsueb 2017). Lipids and carbohydrates are also claimed by some studies as a concentrated source of energy, which is 9.44 kcal g−1 or 39.5 kJ g−1 (Miles & Chapman 2006, Zhou et al. 2007, Hixson 2014, Molina-Poveda 2016, Qiu et al. 2016). Lipids play an important role in the growth performance of P. vannamei, where growth performance is better when fed a 90 g kg−1 diet with lipids at 3 psu than the shrimp that grow at 25 psu (Qiu et al. 2016). This study showed that the shrimp needs more energy during low salinity conditions for osmoregulation. Another study that used the same juvenile shrimp from Zhou et al. (2007) reported that pollack fish oil and the mixture with higher levels of unsaturated fatty acids (HUFAs) are better utilized by the shrimp compared to pork lad, soybean oil, peanut oil, and rapeseed oil due to higher fatty acid composition.
Vitamins are organic compounds required in small amounts for animal growth, reproduction, health, and body maintenance (Bamforth & Cook 2019). Vitamins constitute 5 to 8% of the cost of a commercial diet (Lall & Kaushik 2021). Vitamins comprise two major groups, which are fat-soluble and water-soluble. Fat-soluble vitamins include vitamins A, D, E, and K. At the same time, the water-soluble group consists of vitamin B6, vitamin C (ascorbic acid), vitamin B12, biotin, choline, thiamin, riboflavin, niacin, panto-thenate, folate, and inositol (Perez-Velazquez 2001). Vitamins are important in diets due to their unique functions, serving as coenzymes to form active enzymes and as catalase for catabolism and anabolism of protein, lipids, and carbohydrates (Lall & Dumas 2022). Fat-soluble vitamins function for most animals’ growth, development, body maintenance, and reproduction (Bamforth & Cook 2019, Lall & Kaushik 2021). He et al. (1992) evaluated the effects of fat-soluble vitamins on P. vannamei. They found that the lack of vitamins A and D significantly retarded growth but did not affect the survival rate. However, vitamin E-deficient shrimp displayed the slowest growth.
Minerals in the diet are also important to aid in cellular activities. Some essential minerals in shrimp diets include copper, zinc, calcium, iron, manganese, iodine, sodium, and selenium (Lall & Kaushik 2021). Adding other additives or supplements, such as organic acid, helps to enhance the immune responses in shrimps (Ng et al. 2015). Chumpol et al. (2018) also reported that the mixture of purple non-sulfur bacteria with commercial shrimp feeds augmented growth performance and immunity. Sivaramasamy et al. (2016) studied the mixing of a probiotic (B. subtilis) with diets and biosynthesized with silver nanoparticles (AgNPs). They proved a better growth performance and disease resistance against vibriosis in shrimps than incorporating the probiotic alone. However, further research is still needed on the effects of different probiotics (singly or in combination) on shrimps.
Microdiets (MDs) for shrimp larvae
White shrimps require a high nutritional value in their feed for better growth and robust immunity during the early stage of development. Live feeds are used as the initial diet for a crustacean larva due to its nutritional composition and suitable size range. However, the bottlenecks of using live feeds are the cost of production and sometimes poor nutritional quality, which make it unsuitable as a replacement for artificial diets to the culturing larvae. Additionally, live feeds can harbor pathogenic bacteria causing diseases (Xie et al. 2010, Liu et al. 2022). Moreover, the lack of protein, poor palatability, insufficient supplementation of enzymes, and the presence of anti-nutrients can affect the growth and disease resistance of white shrimp larvae (Wang et al. 2019). Initial developmental stages of shrimp are frequently associated with low optimal growth and survival rates due to cannibalism and reduced disease resistance to opportunistic pathogens (Barreto et al. 2023). These are also associated with shrimps that lack an adaptive immune response and depend uniquely on their innate immune system against pathogenic bacteria, which can result in high mortality and significant economic losses for production (Shinn et al. 2018). Therefore, the aquatic feed industry pays more attention to enriched microdiets as a complete replacement for live feeds.
The benefits of using MDs are balanced nutrition, lower production cost, good palatability, good buoyancy, low dissolution, and low leaching losses (Pedroza-Islas et al. 2004, Hoseinifar & Zare 2013, Liu et al. 2022). A microdiet is a formulated, inert, or first-prepared dry diet for feeding larvae. The size usually ranged between 150 and 800 µm (Kolkovski 2013). There are various routes of administration of microdiets (microencapsulated, microcoated, and micro-bound) to the postlarvae of shrimps, especially P. vannamei (Amaral 2018, Liu et al. 2022). However, microdiets have advantages and disadvantages (Table 3).
Table 3 The definition of microencapsulated, microbound, and microcoated diets, including their advantages and disadvantages.
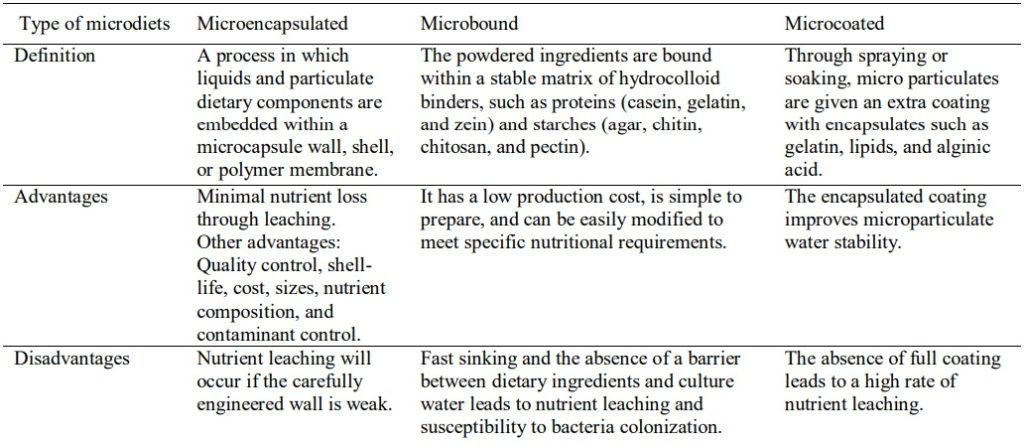
Many studies demonstrated the effects of microdiets on shrimp larvae on either growth or to determine their suitability to replace commercial dry feeds and live feeds. Medina-Reyna et al. (2005) demonstrated that whey protein is a good protein source and wall-forming agent for microencapsulating shrimp larval diets. The results showed that microencapsulated diets supplemented with live algae (Chaetoceros muelleri) on the zoea larvae of white shrimp significantly augmented the survival rate compared to the control diet. Munaeni et al. (2014) revealed that microencapsulated symbiotic treatment (Bacillus sp., whey, and maltodextrin) enhanced the survival rate, feed conversion ratio (FCR), specific growth rate (SGR), immune responses, and disease resistance against V. harveyi in the larvae of P. vannamei. Xie et al. (2010) used a gelatin-walled microencapsulated diet to replace live feed for larval shrimp. The diet significantly increased the wet weight of larval shrimp by 300% during a 30-day growth trial. Singh et al. (2022) supplemented 3% of white button mushroom (Agaricus bisporus) into a microdiet. They recorded significant differences in weight gain, SGR, FCR, protein efficiency ratio, and survival of postlarvae P. vannamei after a 60-day trial, is due to the presence of beta-glucan and antioxidants in the white button mushroom, which is much needed for growth and promoting disease resistance. Microbound formulated diets have also been proven to increase the growth performance of PZ2 or M1 of P. vannamei and can potentially replace Artemia nauplii as a primary feed (Patterson et al. 2016).
Additionally, Liu et al. (2022) demonstrated that the large yellow croaker (Larimichthys crocea) larvae had better growth performance and survival rate when fed with chitosan-coated microdiets. Chitosan is a biocompatible polymer with appropriate wall material for preparing microcoated diets to deliver nutrients to the host. Besides, chitosan also acts as an immunostimulant, which proves the benefits of microbound diets on finfish. In addition, co-feeding microdiets with live feed can replace commercial feeds. Jaseera et al. (2021) showed that co-feeding microdiets with microalgae (Aurantiochytrium sp.) with Artemia as the vector of delivery enhanced the growth performance, survival, and nutritional quality (metabolic enzymes and fatty acid profile) of P. monodon. The feeding of a commercial inert microdiet with Artemia was proven more efficient than the control (without Artemia) in the growth trial of white shrimp (P. vannamei) postlarvae (Amaral 2018). Moreover, Qui et al. (2016) demonstrated that 4% of Schizochytrium meal as an additive in a microdiet improved the growth performance of shrimp larvae.
Microdiets with probiotics for enhancing growth and disease resistance in the white shrimp
Several studies proved that mixing probiotics with feed significantly affected growth, survival, and immune responses against diseases in P. vannamei. Mulyadi et al. (2022) showed that the combination of Lactobacillus sp. and turmeric flour (Curcuma longa) in feed significantly improved survival rate, FCR, absolute growth rate, and feed efficiency ratio in the juveniles of white shrimp. Hien et al. (2022) demonstrated that the postlarvae of P. vannamei improved their growth rate, survival, and immune responses against V. parahaemolyticus when provided a microdiet mixed with a probiotic (Pro-A). The supplementation of Haematococcus pluvialis into microdiets improved the survival and immune capacity in the postlarvae of white shrimp (Xie et al. 2018). Wa Yao et al. (2021) proved that spraying synbiotics onto diets significantly improved the growth performance, nonspecific immunity, and disease resistance of P. vannamei compared to mixing the symbiotic directly into the feed. Huang et al. (2022) demonstrated that a plant extract from Yucca schidigera (Micro-Aid) enhanced growth performance, disease resistance, and gut microbiota of crustaceans. Apart from that, the oral administration of water-soluble multi-strain probiotics (including Bacillus bifidium, Lactobacillus acidophilus, Lactobacillus casei, and Enterococcus faecium) enhanced the growth, feed utilization, digestive enzymes, immune-related genes, and survival of the Pacific white shrimp postlarvae (Miandare et al. 2016). One investigation proved that dietary supplementation of acidic calcium sulfate (Vitoxal) improved the Pacific white shrimp’s stress indicators and immune responses in a 35-day feeding trial (Anuta et al. 2011). Dietary supplementation with chitosan and chitin derived from live feed improved the disease resistance of the white shrimp against vibriosis (Cheng et al. 2021). An antimicrobial peptide (0.4%) has also been used as a supplement with feed to enhance the growth performance, innate immunity, and disease resistance in the Pacific white shrimp against V. harveyi as a potential alternative to antibiotics (Gyan et al. 2020).
In conclusion, the culture of P. vannamei faces significant challenges due to the high mortality rates caused by bacterial and viral diseases. Probiotics have emerged as a promising solution to enhance shrimp health and disease resistance. Various probiotics, including Bacillus, Lactobacillus, and yeast, have positively impacted shrimp growth, immune response, gut microbiota, and water quality parameters. Probiotics in shrimp culture offer a safer alternative to antibiotics, which can adversely affect animals and the environment. However, further research is needed to optimize feeding techniques incorporating probiotics and commercial feed to maximize disease resistance in penaeid shrimp culture.
Shrimp aquaculture is a crucial industry for global fishery and aquaculture farming. However, it faces numerous challenges, including disease outbreaks, poor management practices, and environmental degradation. Diseases such as AHPND, WSD, and vibriosis have caused significant mortality in penaeid shrimp farms, leading to substantial economic losses. Applying probiotics in shrimp culture has shown promise in enhancing disease resistance. Probiotics, such as Bacillus and Lactobacillus, have improved growth performance, immune response, and disease resistance in shrimp. Probiotics offer a cost-effective and environmentally friendly alternative to antibiotics, which have limitations and can contribute to developing drug-resistant bacteria. Furthermore, advanced research is needed to optimize the administration and formulation of probiotics in shrimp feeds for maximum efficacy.
Commercial feeds, particularly fish meal, play a vital role in providing the necessary nutrients for the growth and development of penaeid shrimp. However, unbalanced nutrient cycles and deficiencies in some commercial diets can lead to poor water quality and increased disease susceptibility. To address these issues, additives such as organic acid blends have shown promise in reducing shrimp mortality when challenged with pathogenic bacteria. The nutritional requirements of penaeid shrimp include proteins, lipids, vitamins, minerals, carbohydrates, and energy. Ensu-ring that commercial feeds meet these nutritional requirements is essential for maintaining shrimp health and disease resistance. Further research is needed to develop optimized commercial feeds that provide balanced nutrition and incorporate probiotics to enhance disease resistance in penaeid shrimp culture.
Source : Open access : Haris, Danial Iman, Yaminudin, Jasmin, Othman, Siti Hajar, Lim, Keng Chin, Ismail, Illisriyani, Wulan Sari, Putri Desi, & Karim, Murni. (2024). The use of commercial feed and microdiets incorporated with probiotics in Penaeid shrimp culture: a short review. Latin american journal of aquatic research, 52(3), 350-367. https://dx.doi.org/10.3856/vol52-issue3-fulltext-3146